Preface
String Time Crystals and Axion Time Crystals are theoretical constructs that push the boundaries of our understanding of time and its symmetries. Leveraging the framework of string theory and considering novel particles like axions, illuminates new aspects of temporal order and chaos. The potential to unify these concepts with cosmological phenomena makes this an exciting area of research, one that promises to deepen the understanding of both the microcosmic and macrocosmic aspects of the universe.
Chapter 1: Introduction
Axion Time Crystals represent a new frontier. Inspired by recent advancements in string theory and time crystals, the formulation within the framework of strings explores how the additional dimensions and energy dynamics in string theory can offer novel insights into periodic structures in time. The potential of string-theoretical models expands the understanding of time’s crystalline behavior. Periodic motion is a fundamental aspect of our universe, observable everywhere, from atomic systems to the celestial orbits of planets. In classical physics, periodicity in space has led to the formation of space crystals—solid structures that break spatial translation symmetry. Time Crystals, as originally proposed, are the temporal analog of these spatial structures. String Time Crystals integrate the principles of string theory into the study of time-periodic systems.
String Time Crystals draw on string theory’s portrayal of fundamental particles as one-dimensional vibrating strings. In this view, the idea of spontaneous temporal symmetry breaking gains an added layer of complexity, as strings can vibrate in higher-dimensional spaces and interact with additional degrees of freedom. These oscillations can lead to novel, stable temporal patterns that mirror the properties of spatial crystals, extending the original work of Shapere and Wilczek to a more multidimensional framework.
Chapter 2: Spontaneous Breaking of Space and Time Symmetry
In String Time Crystals, spontaneous breaking of symmetry happens in both space and time. In higher-dimensional space, strings can exhibit both spatial and temporal periodicity, leading to the formation of complex crystalline structures not just in conventional 3D space but across additional dimensions predicted by string theory. The spontaneous emergence of such periodic structures is due to the unique dynamics and interactions that strings can have, compared to point particles.
Chapter 3: String Time Crystals – Formation and Characteristics
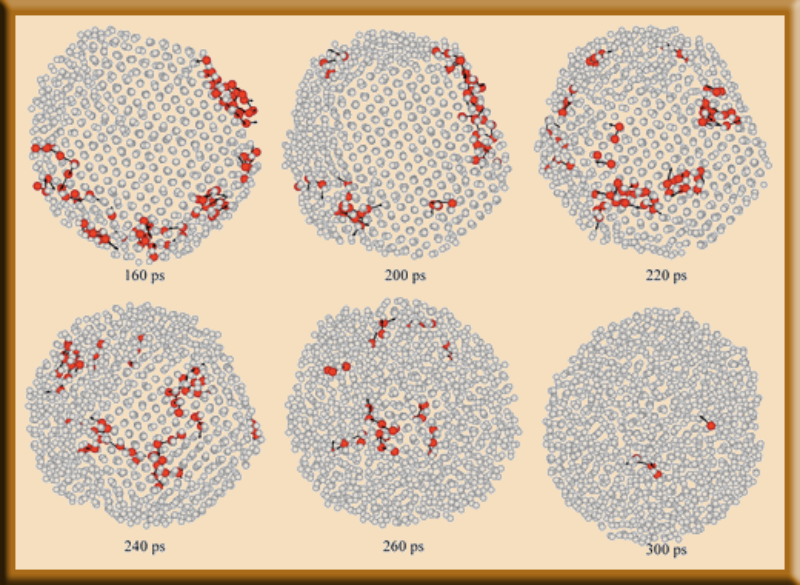
String Time Crystals arise from string-theoretical dynamics. By considering multi-dimensional oscillatory behavior and the influence of compactified dimensions, it is explored how strings can organize themselves into repeating temporal patterns. Recent studies have established that collective atomic motion, particularly string-like patterns observed during the melting and freezing of nanoparticles, plays a crucial role in the formation of temporal structures [1]. These string-like cooperative motions, originally found in glass-forming liquids and grain boundaries, suggest that the emergence of periodic structures is a fundamental property of interacting particle systems. Additionally, strained crystalline nanomechanical resonators have demonstrated significantly lower mechanical dissipation due to dissipation dilution, which combines tensile stress with geometric nonlinearity, achieving quality factors exceeding at cryogenic temperatures [2]. These findings open new possibilities for enhancing the precision of quantum technologies and for connecting these crystalline structures to the cosmological phenomena of the early universe.
Chapter 4: Discrete String Time Crystals and Related Phenomena
Discrete String Time Crystals involve periodic driving and external forces that cause strings to break temporal symmetry in discrete time intervals. This chapter includes the mathematical formalism needed to describe such crystals, including Floquet theory adapted for higher-dimensional vibrating strings. We discuss recent experiments and simulations that suggest the feasibility of realizing discrete String Time Crystals in laboratory settings involving quantum simulators and photonic lattices.
Chapter 5: Axion Time Crystals
Axion Time Crystals are based on the hypothetical axion particle—a candidate for dark matter that interacts very weakly with ordinary matter. Axions, due to their particular properties, are theorized to form coherent fields that can oscillate, effectively behaving like a time crystal. This chapter explores how axion fields, under certain conditions, can lead to stable temporal patterns. These patterns have been shown to be achievable using synthetic materials such as spacetime crystals, where spacetime modulation provides a means to realize an enhanced axion response. The use of glide-rotation symmetry in such spacetime crystals results in nonreciprocal couplings that significantly amplify the axion-like behavior, making it several orders of magnitude larger compared to naturally occurring materials like Cr₂O₃ [3]. This synthetic axion response is interesting not only for its practical applications in optics—such as electromagnetic isolation and novel light-wave interactions—but also for its conceptual link to high-energy physics and its potential role in understanding dark matter.
The interaction between axions and electromagnetic fields, as well as their coupling to strings, offers an exciting avenue for studying new kinds of temporal symmetries and their breaking. By investigating the interplay between axions and String Time Crystals, potential mechanisms for observing these phenomena experimentally are proposed, possibly offering insights into the elusive nature of dark matter.
The interaction between axions and electromagnetic fields, as well as their coupling to strings, offers an exciting avenue for studying new kinds of temporal symmetries and their breaking. By investigating the interplay between axions and String Time Crystals, we propose potential mechanisms for observing these phenomena experimentally, possibly offering insights into the elusive nature of dark matter.
Chapter 6: Realizations and Future Directions
Existing laboratory techniques, from ultracold atoms to superconducting qubits, can be adapted to the higher-dimensional and exotic requirements of string-based models. It is well established that collective atomic motion plays a critical role in phase transitions, particularly in systems such as nanoparticles, where melting and freezing are accompanied by string-like cooperative atomic motion [1]. This motion, initially observed in the interfacial dynamics of glass-forming liquids and grain boundaries, suggests that string-like structures emerge naturally during phase changes, propagating from surfaces and interfaces. Additionally, strained crystalline silicon resonators have shown ultralow mechanical dissipation with quality factors surpassing at 7 K, thanks to dissipation dilution mechanisms [2].
Recent advances in synthetic axion materials offer new avenues for realizing enhanced nonreciprocal responses, such as those seen in spacetime crystals. These synthetic materials employ spacetime modulation and glide-rotation symmetry to achieve giant axion-like behavior, which has implications for precision measurement and quantum sensing technologies. The implications of these new types of time crystals for future technologies, such as quantum computing, electromagnetic isolation, and nonreciprocal light-wave interactions, are significant and are directly related to the fundamental questions of time, entropy, and the nature of the universe [3].
Conclusion
The study of Axion and String Time Crystals opens a new dimension in our understanding of temporal order, symmetry breaking, and the underlying fabric of the universe. By leveraging string theory and considering exotic particles like axions, these constructs provide a deeper insight into both the microcosmic properties of quantum systems and the macrocosmic evolution of the cosmos. The spontaneous breaking of temporal symmetry and the formation of stable temporal patterns show how complex, ordered behavior can emerge from fundamental physical interactions, bridging theoretical physics with observable phenomena. The potential experimental realizations, ranging from quantum simulators to photonic lattices, bring these theoretical predictions closer to empirical validation. Moreover, the insights gained from these studies could have significant implications for advancing future technologies, such as quantum computing and precision measurement. As we continue to investigate these intriguing constructs, we may uncover deeper connections between quantum field theory, dark matter, and the nature of time itself—paving the way for breakthroughs in understanding the universe’s most elusive aspects.
References
[1] Zhang, H., Kalvapalle, P., & Douglas, J. F. (2011). String-like collective atomic motion in the melting and freezing of nanoparticles. Journal of Physical Chemistry B, 115(45), 14068-14076. https://doi.org/10.1021/jp203765x
[2] Ghadimi, A. H., Fedorov, S. A., Arabmoheghi, A., Bereyhi, M. J., Schilling, R., Wilson, D. J., & Kippenberg, T. J. (2021). Strained crystalline nanomechanical resonators with ultralow dissipation. Nature Communications, 12, Article 2118. https://doi.org/10.1038/s41467-021-22431-1
[3] Prudêncio, F. R., & Silveirinha, M. G. (2022). Synthetic Axion Response with Spacetime Crystals. arXiv preprint arXiv:2209.03314. https://arxiv.org/abs/2209.03314